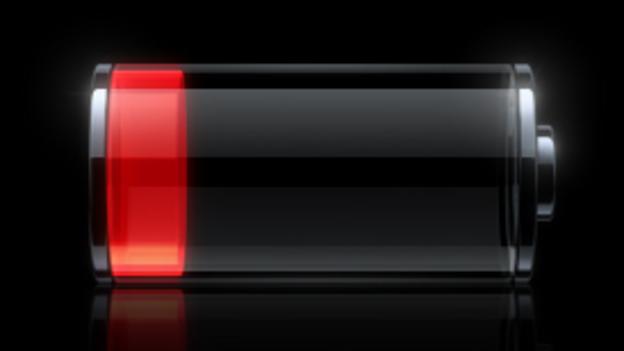
(Copyright: Apple)
In labs around the world, scientists are looking for ways to power up your phone and, once again, allow mobiles to live up to their name.
It is a familiar problem. You pull your glossy smartphone from your pocket to make a call but there are no signs of life. How can that possibly be? You plug it in every night. You are careful not to spend too long looking at Facebook. You hardly ever use the GPS and you always wait until you are on your laptop at home to click on those YouTube links. But still, here you are, clutching a “phone” with a dead battery.
The truth is that the power demands of our feature-filled smartphones have outpaced any improvements in battery technology. And it is only going to get worse as next-generation 4G networks come online, giving phones access to high-speed always-on connections and torrents of data. Without a step change in battery technology the digital nomads of tomorrow will be paralyzed - tied to the plugs and cables these new tools were supposed to banish.
"I don't think we are too far from the point when batteries, if they don't evolve more quickly, are going to create a noticeable drag on what your smartphone can offer,” says Natasha Stokes, editor of Mobile Choice magazine,. Without a radical response, she predicts that it will not be long before power hungry, feature rich phones last just “six hours”.
There are various nascent technologies looking to solve the problem, such as solar panels embedded in the screen or kinetic devices that harness your movements to charge a handset. But, these are a long way off from offering a practical solution. What is needed is an entirely new type of battery, say researchers.
And now, help could be on its way. In labs around the world, teams are testing new materials, chemistries and technologies that aim to supercharge your phone and, once again, allow mobiles to live up to their name.
Memory trick
Batteries are all largely based on the same simple principle: they convert chemical energy into electrical energy. To do this, they contain a positive and a negative electrode – known as a cathode and anode - separated by a substance known as an electrolyte. When these electrodes are connected to a circuit, a series of chemical reactions is kicked off. At one end, charged particles from the electrolyte – known as ions - flow to the anode, react and release electrons. At the other end, reactions at the cathode create a material that – like a sponge - wants to suck up these free electrons.
The result is a system brimming with electrons at the anode that want to move to the cathode. But this is where the electrolyte’s second job comes on - it prevents the electrons taking the direct path, instead forcing them through the attached circuit – say the wiring to a light. It is this flow of electrons through the electric circuit that creates the electric current. In rechargeable batteries the reactions are reversible, with the ions and electrons flowing back in the opposite direction during charging.
This same sort of reaction is at the heart of the rechargeable lithium-ion batteries used in almost every smartphone. These have an anode made of carbon (usually graphite) a metal oxide cathode and an electrolyte containing lithium salt. Lithium has become the metal of choice for these batteries because it is relatively easy to split ions from lithium metal, triggering the important reactions and boosting performance. The technology has been around since the 1970s but it was not until the introduction of the first commercial units by Sony in 1991 that they really took off, replacing its nickel cadmium predecessors. The market for lithium-ion power packs is now worth more than $12bn and is set to rise to nearly $54bn in 2020.
Their dominance is down to several reasons – they are lightweight; they tend to hold their charge better than other batteries and they do not suffer from so-called “memory effect”, where batteries hold less and less charge if they are not drained and then recharged completely. Critically, they also have a high energy density - a measure of how much punch a battery packs.
Over the last 20 years, researchers and manufacturers have managed to continually squeeze more and more energy out of these packs, doubling their performance. This has been achieved by clever engineering, subtly tweaking the structures inside the battery to make them more efficient, or adding new materials to boost their performance. And the process carries on today, with materials such as silicon receiving a lot of interest as a possible replacement – and improvement - for the graphite anodes in lithium-ion batteries.
Silicon is attractive because it is cheap, abundant and well understood. But more importantly, by weight, it can store ten times more lithium ions than graphite, which means that it could theoretically allow a 10-fold increase in performance. However, to be useful, researchers must overcome a problem. While graphite anodes hold their shape when they soaks up lithium ions, silicon swells, causing silicon particles to become separated, rapidly reducing the performance of the battery.
To try to get around this, Dr Gao Liu, of the Lawrence Berkeley National Laboratory in Berkeley, California, is developing rubbery conductive binders that stick to the silicon particles within the anode, stretching and shrinking as the battery is charged and discharged. He worked with theoretical chemists to identify suitable materials for these restraints, finally settling on a conductive plastic. Initial results suggest his new anode could produce lithium-ion batteries with 25 to 30% greater capacity and longer life span than those on the market today.
"Our latest tests have shown our material works well and is really stable," says Dr Liu. "It keeps its conductivity, structure and capacity well even after more than 1,000 charging cycles." He is collaborating with commercial partners including the multinational 3M and says he is currently taking phone calls "almost every day" from consumer electronics companies.
But he will have to act quickly. US companies Amprius and Nanosys, are already developing lithium-ion batteries with anodes containing silicon nanowires to get around the swelling issue, whilst electronics giant Panasonic is due to launch lithium-ion laptop batteries with silicon alloy anodes, which it says boosts capacity by 30%. The firm has not announced a mobile phone battery but industry watchers suggest one may be in development.
But, despite the commercial interest, these advances will soon reach a bottleneck. At best, these incremental improvements will performance, researchers say.
“The lithium ion research field is 30 years old now and commercialisation is over 20 years old,” says Prof Gerbrand Ceder of the Massachusetts Institute of Technology (MIT) in US. “It's hard as a scientist to say something isn't possible but when you look at the candidates it doesn't look as if we're going to get dramatically better capacity which is why we are starting to see a lot of research on totally different chemistries and totally different technologies."
Short circuit
One example is lithium-air batteries, inspired by the zinc-air packs first used in hearing aids in the 1960s that get their power by reacting zinc with oxygen in the air. Professor Peter Bruce, of the University of St Andrews, Scotland, has been working on the idea since 2007, with anodes made of lightweight porous carbon. Oxygen from the air enters the porous carbon, reacts with the lithium ions in the electrolyte and electrons in the external circuit to form solid lithium oxide. Recharging causes the lithium compound to decompose, releasing the lithium ions and releasing the oxygen.
Calculations of the amount of energy involved in the chemical reactions involved suggest it could produce batteries that last three to five times as long as existing lithium-ion ones – hinting at a day when your smartphone could once again hold a working week’s worth of charge. However, Prof Bruce says much work is still needed, particularly in identifying stable electrolytes that only allow useful electrochemical reactions without wasteful additional reactions.
Meanwhile other groups in the US, Europe and Asia have joined the race. "It's an exciting time, but there are still considerable challenges remaining," says Prof Bruce. “The science is promising but we can't yet guarantee it will end in a workable technology."
But it is not the only lithium-based battery looking to sneak into your next phone. Researchers are also investigating light weight lithium-sulfur packs, which are likely to have a life span of three times that of current lithium-ion batteries. These were first touted in the 1940s and use one electrode made from sulfur and another from lithium. They are already used in specialist communications and military applications but, despite high hopes and high-profile displays of the technology, they have never managed to hit the mainstream. In part, this is because they have had inherent problems such as losing their ability to recharge efficiently after a relatively short space of time and safety issues which mean the metal can melt and potentially catch fire if it comes into contact with water.
However, Sion Power, of Tucson, Arizona, says it has overcome these problems. It has a prototype with around 50% more energy capacity by weight than existing lithium-ion batteries, and says it plans to target markets including mobile phones and notebooks. In 2010 it was awarded a grant worth up to $5 million from the US government to develop safe, practical and economically viable rechargeable lithium-sulfur batteries in three years.
The firm refuses to give specific details about the technology it uses but says it has dealt with the safety issues. These are known to be caused when branch-like growths grow on the lithium metal electrode, causing it to heat up and potentially short-circuit. Prototypes seen elsewhere suggest that they may have accomplished this by treating the electrodes to stop the growths and adding plastic or ceramic membranes to separate the electrodes and prevent short circuits. The company says it has also made progress with the recharging problem. It has prototypes that keep their capacity over 50 cycles but has claimed it is hopeful of reaching 1,000 cycles soon – the equivalent of today’s lithium-ion batteries.
But some firms and researchers argue that despite intense research and millions of dollars of investment, both of these lithium based technologies are far from delivering on their promise. As a result, MIT’s Professor Ceder is taking a more radical approach. He has used high-performance computers to create a public database of around 20,000 chemical compounds. A series of algorithms predict the properties of these materials, allowing researchers to quickly model how two compounds would react with each other in a battery.
“The kind of properties we're talking about are the kind of battery voltages possible with compounds, the mobility of ions and electrons in materials, chemical stability, safety, also indications about how the materials might be made,” he says. The Materials Project, as it is known, has already been used to identify three new materials with the potential to be used in batteries. A lot more work needs to be done on these, he said, but his computational approach is already paying off in work on another technology known as magnesium-ion batteries.
These use a magnesium metal anode and could have two to three times the energy density of the best lithium-ion batteries. In addition, tests have shown they hold the majority of their charge over 3,000 charge cycles, many more than today’s smartphone batteries. Magnesium is also cheap and abundant. However, the technology is still unproven. To be truly useful, researchers need to find a suitable cathode, something that has held back previous efforts. And this is where his computational approach comes in.
Prof Ceder and his colleagues have virtually screened more than 12,000 materials. Those that show potential are synthesized and tested. At least four materials are now being studied in detail. He claims that one cathode material has already demonstrated performance that would create batteries with greater energy density than today's lithium ion technology. He will not reveal more details, but it is enough to have attracted significant interest from investors and the US military. He has also established a start-up called Pellion Technologies to exploit the technology, initially for use in cars. However, if he is successful the technology could trickle down to mobile phones in years to come.
Apple juice
If and when it does, it may have to compete with another powerful contender for your future phone – the fuel cell. Like batteries, these convert chemical energy into electricity through a chemical reaction. However, these work more like tiny engines, converting the chemical energy from a fuel – an alcohol, say - into electricity through a chemical reaction with oxygen. And like an engine, they continue to run providing there is enough fuel and oxygen flowing through them.
They are an old technology dating back to the 19th Century and were successfully used to power elements of the early Gemini and Apollo space missions, for example. But they have now caught the attention of phone manufacturers because they have a high energy density. Simply put, fuels contain lots of energy. For example, hydrogen contains nearly 150 times the energy of an equivalent weight of lithium. However, to be workable they need to be small and have an easily rechargeable reservoir for fuel.
To build these, researchers are focusing on so-called MEMs technology - miniaturized mechanical devices and structures built using the patterning and etching techniques pioneered to build early computer chips. These are already used to make everything from solar cells to flat-screen TVs. Crucially, these techniques have advanced to the point where they can now be used to construct complex 3D structures – such as the networks of channels and pipes necessary for a tiny fuel cell.
The technology can be expensive, with precious metals such as platinum and palladium used to speed up chemical reactions, and hydrogen sources, even when compressed or in liquid form, taking up a lot of space. There are also the practicalities of having to moving and storing the fuel necessary to charge your phone on the go, not to mention the potential problems of handling potentially toxic fuels to make a phone call.
However, companies such as NEC and Toshiba have already shown off prototype fuel cells for mobiles. And in December 2011, news that Apple had filed two more patent applications for fuel cells for portable electronic devices reignited interest. The applications showed the company is proposing the use of reducing agent sodium borohydride, which when mixed with water generates pure hydrogen, and that they would be used alongside a battery to cut down on size, weight and cost. The documents suggest the technology could power devices for "days or even weeks".
But, according to St Andrew’s Prof Bruce, we should not get too excited yet. “In the longer term there are these radical technologies which could transform energy storage for consumer electronics applications,” he says. But, for the next few years, he believes, lithium-ion batteries are here to stay. Better remember to pack that charger.
0 comments:
Post a Comment